Quantum nanoscience
Quantum nanoscience is the basic research area at the intersection of nanoscale science and quantum science that creates the understanding that enables development of nanotechnologies. It uses quantum mechanics to explore and use coherent quantum effects in engineered nanostructures. This may eventually lead to the design of new types of nanodevices and nanoscopic scale materials, where functionality and structure of quantum nanodevices are described through quantum phenomena such as superposition and entanglement. With the growing work toward realization of quantum computing, quantum has taken on new meaning that describes the effects at this scale. Current quantum refers to the quantum mechanical phenomena of superposition, entanglement and quantum coherence that are engineered instead of naturally-occurring phenomena.
Fundamental concepts
Coherence
Quantum nanoscience explores and uses coherent quantum effects in engineered nanostructures. Coherence is the property of a quantum system that allows to predict its evolution in time, once it has been prepared in a superposition of different quantum states. This property is important when one intends to use the system for specific tasks, such as performing a sequence of logic operations in a quantum computer. Quantum coherence is fragile and can easily be lost if the system becomes too large or is subjected to uncontrolled interactions with the environment. Quantum coherence-enabled functionality holds the promise of making possible disruptive technologies such as quantum computing, quantum communication, quantum simulation, and quantum sensing. Coherent quantum effects at the nanoscale are relatively uncharted territory. Therefore, the field of quantum nanoscience is special among basic sciences because it provides a pathway into this frontier of human knowledge.
Quantum coherence is at the very heart of quantum nanoscience. The goal of the field is to manipulate and exploit quantum-coherent functionality. Much of the quantum nanoscience is dedicated to understanding the mechanisms of decoherence in order to preserve and maximize coherence.
Superposition
Superposition is the quantum phenomena wherein an entity can simultaneously exist in two states. The classic description is the thought experiment of Schroedinger’s Cat. In this gedanken experiment, the cat can be both alive and dead until the state of the cat is actually observed.
Entanglement
Entanglement can link the quantum states of two or more objects over any distance. Entanglement lies at the heart of quantum teleportation and quantum communication.
Enabling constituents
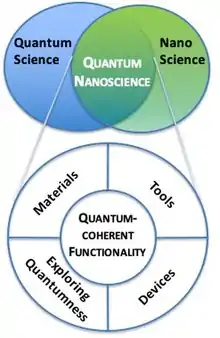
The pursuit of quantum coherence-enabled functionality includes the enabling fields of quantum nanoscientific research, such as enabling materials and tools that are directed towards the goal of achieving coherence-enabled functionality. The elements of quantumness, materials, tools, and fabrication are all quantum and/or nano. Quantum nanoscience can include these as long as they are in pursuit of path toward quantum coherent functionality.
Applications
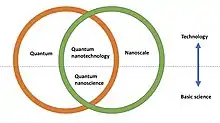
- Quantum computing
- Quantum communication is ultra-secure, hack-proof communication using entangled states.
- Quantum simulator
- Quantum sensing uses a quantum state in order to sense another object. The fragility of coherence can be turned into a resource by using the loss of coherence of the quantum system as a sensitive tool to probe the environment itself.
See also
- Quantum
- Quantum computer
- Kavli Prize - awards for outstanding scientific work in the fields of astrophysics, nanoscience and neuroscience
- Center for Quantum Nanoscience
References
Further reading
- Feynman, Richard P. (March 1992). "There's plenty of room at the bottom [data storage]". Journal of Microelectromechanical Systems. 1 (1): 60–66. doi:10.1109/84.128057. ISSN 1057-7157. S2CID 40094454.
- Benioff, Paul (May 1980). "The computer as a physical system: A microscopic quantum mechanical Hamiltonian model of computers as represented by Turing machines". Journal of Statistical Physics. 22 (5): 563–591. Bibcode:1980JSP....22..563B. doi:10.1007/BF01011339. ISSN 1572-9613. S2CID 122949592.
- Benioff, Paul (7 June 1982). "Quantum Mechanical Models of Turing Machines That Dissipate No Energy". Physical Review Letters. 48 (23): 1581–1585. Bibcode:1982PhRvL..48.1581B. doi:10.1103/PhysRevLett.48.1581.
- Eigler, Donald; Schweizer, Erhard K. (5 April 1990). "Positioning single atoms with a scanning tunnelling microscope" (PDF). Nature. 344 (6266): 524–526. Bibcode:1990Natur.344..524E. doi:10.1038/344524a0. S2CID 4323687. Retrieved 25 January 2019.
- Milburn, Gerard J.; Woolley, M. J. (2008). "Quantum nanoscience". Contemporary Physics. 49 (6): 413–433. Bibcode:2008ConPh..49..413M. doi:10.1080/00107510802601724. S2CID 218544375.
- Seeman, Nadrian (23 January 2003). "DNA in a material world" (PDF). Nature. 421 (6921): 247–231. Bibcode:2003Natur.421..427S. doi:10.1038/nature01406. PMID 12540916. S2CID 4335806. Retrieved 25 January 2019.
- Gerard J. Milburn, Paul Davies, Schrödinger's Machines: The Quantum Technology Reshaping Everyday Life (ISBN 0716731061)
- Deutsch D., Physics, Philosophy, and Quantum Technology, in the Proceedings of the Sixth International Conference on Quantum Communication, Measurement and Computing, Shapiro, J.H. and Hirota, O., Eds. (Rinton Press, Princeton, NJ. 2003)
- V.E. Tarasov, Quantum Nanotechnology, International Journal of Nanoscience. Vol.8. No.4-5. (2009) 337—344.
External links
- Department of Quantum Nanoscience - Kavli Institute of Nanoscience
- Quantum Nanoscience Group - The Australian Research Council Nanotechnology Network
- Center for Quantum Nanoscience
- Mike and Ophelia Lazaridis Quantum Nanoscience Center
- Quantum Nanoscience Division - Peter Grünberg Institute, Research Center Jülich