Particle receiver
A particle receiver is an object placed on the top of a solar tower on which surface solar energy is concentrated by means of a solar field composed of large number of mirrors, called heliostats. The goal is to transform solar energy into thermal energy that can be used in a heat process, thermochemical process, or in a heat engine to produce electricity in a solar tower power plant. To accomplish this, it is necessary to introduce certain material, called heat transfer medium, to the receiver that is then heated up, either directly or indirectly, by the concentrated solar energy before leaving the receiver at a higher temperature. Unlike receivers used in conventional concentrated solar power (CSP), power plants which employ molten salts as a heat transfer medium that is heated indirectly by flowing through the metal tubes that are exposed to the concentrated solar energy, particle receivers adopt solid particles which then can be heated either directly or indirectly, depending on the technology considered.[1][2]
.jpg.webp)
One of the main advantages of adopting particles as a heat transfer medium is the possibility of direct heating, where particles are exposed directly to the incoming solar radiation, thus avoiding issues related to non-uniform heating of receiver tubes.[3] Also, the possibility to reach temperatures above 1000°C[1] allows for the adoption of Brayton cycle with supercritical CO2 as the working fluid which can achieve higher thermal efficiency compared to the steam Rankine cycle which is used in the conventional CSP power plants that have a maximum temperature limit of 565°C due to issues related with the thermal stability of the molten salts.[2][4][5]
Directly heated particle receiver
Free falling receiver
.jpg.webp)
This technology is based on a free falling particle curtain inside the receiver cavity that absorbs concentrated solar radiation. Idea of using falling solid particles in a concentrated solar power facility to supply high temperature heat to the power cycle or chemical process was introduced in a pioneering work carried out by Martin and Vitko during the beginning of the 1980s at Sandia National Laboratories.[6][7] However, first step towards demonstrating the concept at the larger scale was carried out in 2009 at National Solar Thermal Test Facility in Albuquerque, NM where prototype particle receiver was placed atop the 61 meter tall solar tower with a solar field able to provide 5 MWth. These tests resulted in receiver thermal efficiency of around 50% and maximum temperature increase of the particles of around 250°C.[8] More comprehensive tests were carried out in 2015 using a 1 MWth receiver having a 1 by 1 meter aperture through which concentrated sun radiation enters the cavity. Receiver thermal efficiency ranged from 50% to 80% and temperature of the particles at the bottom of receiver reached 700°C in some cases.[9]
Limit on the maximum temperature of molten salts used in the conventional solar tower power plants led to a workshop organized by United States Department of Energy (DOE) in August of 2016 which identified three possible pathways for the next generation CSP power plants based on the following heat transfer carriers: molten salts, solid particles, and gaseous fluids.[2] This further led to the Generation 3 Concentrating Solar Power Systems funding program that started on May 15 of 2018 when DOE announced its intention to provide $72 million to the project where three teams are going to compete in building a system integrated with a thermal energy storage that is able to efficiently capture solar energy and provide it to the working fluid of the power cycle at temperatures above 700°C.[10] On March 25, 2021 DOE announced that the pathway adopting falling solid particles is the most promising one for achieving 2030 cost targets of 0.05$/kWh and awarded Sandia National Laboratories with $25 million for building, testing, and operating pilot plant adopting particle receiver at National Solar Thermal Test Facility which is expected to be completed by the end of 2024.[11][12]
Obstructed flow receiver
Idea of obstructing the flow of particles while retaining the concept of direct heating is motivated by the fact that by slowing down the flow of particles, it is possible to increase thermal efficiency of the receiver by increasing the opacity of the particle curtain and reduce their loss through the aperture. Early tests of this concept were carried out at Sandia during the 1980s, but no analytical nor experimental studies were published until 2010s. Experiments carried out at Sandia in 2015 using chevron-shaped porous structures managed to improve heating of the particles and reduce their loss through the receiver aperture. However, there were problems related to the direct exposure of the stainless steel 316, used for constructing these porous structures, to concentrated solar radiation, and its wear due to particle flow over it.[1][9] Another design proposes to use a spiral ramp over which particles flow due to the combined effect of the gravitational force and mechanically induced vibrations. Tests showed that it is possible to reach particle temperature of 650°C and thermal efficiency of around 60%. However, this design requires beam down optics that would result in additional optical losses and significant particle flow could be difficult to obtain using the proposed design.[13]
Centrifugal receiver
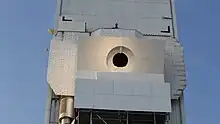
This concept is based on a rotating cylindrical cavity that can be inclined with respect to the horizontal direction. Due to rotation of the cavity, particles form thin but opaque layer over the cavity inner surface. These particles are then heated by concentrated solar radiation while they descend slowly along the axial direction of the cavity due to effect of the gravitational force.[14] Concept was proposed initially by Flamant in the late 1970s and early 1980s but there were no further developments until DLR started working on this concept in the early 2010s when they started with laboratory scale prototype testing.[15] Proof-of-Concept 15 kWth centrifugal receiver was designed, built , and tested at the DLR facility in Cologne and the results showed that it is possible to reach outlet particle temperatures of over 900°C. However, due to problems in measuring particle mass flow rate it was not possible to determine receiver thermal efficiency.[14] Further experimental campaigns obtained thermal efficiency of 75% for particle outlet temperature equal to 900°C with an incident heat flux of 670 kW/m2.[16] As of 2018 this receiver concept is installed at Jülich Solar Tower test facility.[17]
Fluidized receiver
This receiver can operate on two possible working principles. First one was proposed by Flamant at the same time when he proposed the centrifugal receiver, and it is based on a transparent silica wall through which concentrated solar energy passes and heats solid particles that are suspended by means of compressed air. Goal was to heat the suspended particles in order to perform decarbonation of CaCO3 an therefore the conversion of solar energy to the thermochemical one. Tests carried out showed that it is possible to reach particle temperatures above 1200°C.[18] Second working principle was proposed by Hunt during late 1970s and it is based on the injection of very small particles inside the stream of compressed air that absorb concentrated solar energy, and due to their large surface area they immediately transfer that heat to the surrounding air. Heating of the mixture is performed until the particles vaporize and then the air is sent to the Brayton cycle to produce electric energy.[19]
Indirectly heated particle receiver
Gravity-driven particle flow through enclosures
This receiver concept is composed of an enclosure with horizontal tubes with their external side inside of the enclosure and having their internal side irradiated with concentrated solar energy. The idea is that particles are going to flow down inside the enclosure due to the gravitational force and around the staggered array of tubes while being heated. Tests showed that heat transfer between particles and tubes was reduced in the areas where particles lost contact with the tubes, however no data regarding the temperatures and thermal efficiencies were reported. Advantages of this concept include absence of particle loss due to the presence of the enclosure, however issues related to the thermal stresses on the enclosure may arise due to indirect heating of the particles.[1]
Fluidized particle flow through tubes
This concept is similar to the one of the directly heated fluidized receiver, only difference is that now tubes are not transparent and particles are heated indirectly by the metal tubes. Flamant proposed and demonstrated this concept and obtained suspension temperatures up to 750°C, however he did not report thermal efficiencies. Possible problems related to this idea include electric energy consumption for the fluidization of particles in the receiver and possible hotspots and high surface temperatures that can increase radiation losses to the environment.[1]
Particle selection
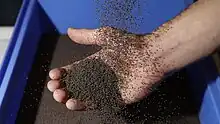
Important factor in evaluating thermal performance of the particle receiver and economic viability of the whole plant is the type of particles used. Desired properties include low cost, high thermal stability, and in case of directly heated receivers, optical properties. Natural materials are considered due to their low price, however attention should also be paid to the composite materials that can be synthesized to enhance desired properties even though they have a higher price. As in directly heated receivers solid particles serve as the solar absorbers, their optical properties become crucial in evaluating receiver performances. It has been shown that increasing solar absorptivity is more important than reducing thermal emissivity, meaning that particles with high emissivity can still be considered as a good candidate if they have high absorptivity, but particles having low absorptivity cannot be considered as a good candidate even if they have low emissivity.[20] Sintered bauxite particles have high solar absorptivity, and it has been shown that despite certain degradation due to prolonged heating, they are able to keep it above 90%.[21] It has also been shown that they are the most durable ones among the other candidate particles.[22] Therefore it has been concluded that they are the best candidate for the use in directly heated particle receivers, and in particular intermediate-density casting media "Accucast" has been deployed at Sanidia's National Solar Thermal Test Facility.[23]
References
- Ho, Clifford K. (2016-10-25). "A review of high-temperature particle receivers for concentrating solar power". Applied Thermal Engineering. Special Issue: Solar Energy Research Institute for India and the United States (SERIIUS) – Concentrated Solar Power. 109: 958–969. doi:10.1016/j.applthermaleng.2016.04.103. ISSN 1359-4311.
- Mehos, Mark; Turchi, Craig; Vidal, Judith; Wagner, Michael; Ma, Zhiwen; Ho, Clifford; Kolb, William; Andraka, Charles; Kruizenga, Alan (2017-01-01). "Concentrating Solar Power Gen3 Demonstration Roadmap". doi:10.2172/1338899. OSTI 1338899.
{{cite journal}}
: Cite journal requires|journal=
(help) - Marugán-Cruz, C.; Flores, O.; Santana, D.; García-Villalba, M. (2016-05-01). "Heat transfer and thermal stresses in a circular tube with a non-uniform heat flux". International Journal of Heat and Mass Transfer. 96: 256–266. doi:10.1016/j.ijheatmasstransfer.2016.01.035. hdl:10016/32068. ISSN 0017-9310.
- Liu, Ming; Steven Tay, N. H.; Bell, Stuart; Belusko, Martin; Jacob, Rhys; Will, Geoffrey; Saman, Wasim; Bruno, Frank (2016-01-01). "Review on concentrating solar power plants and new developments in high temperature thermal energy storage technologies". Renewable and Sustainable Energy Reviews. 53: 1411–1432. doi:10.1016/j.rser.2015.09.026. ISSN 1364-0321.
- Bauer, Thomas; Pfleger, Nicole; Laing, Doerte; Steinmann, Wolf-Dieter; Eck, Markus; Kaesche, Stefanie (2013-01-01), Lantelme, Frédéric; Groult, Henri (eds.), "20 - High-Temperature Molten Salts for Solar Power Application", Molten Salts Chemistry, Oxford: Elsevier, pp. 415–438, doi:10.1016/b978-0-12-398538-5.00020-2, ISBN 978-0-12-398538-5, retrieved 2023-07-04
- Martin, J.; Jr, Vitko J. (1982-01-01). "ASCUAS: a solar central receiver utilizing a solid thermal carrier". doi:10.2172/5663779. OSTI 5663779.
{{cite journal}}
: Cite journal requires|journal=
(help) - Tan, Taide; Chen, Yitung (2010-01-01). "Review of study on solid particle solar receivers". Renewable and Sustainable Energy Reviews. 14 (1): 265–276. doi:10.1016/j.rser.2009.05.012. ISSN 1364-0321.
- Siegel, Nathan P.; Ho, Clifford K.; Khalsa, Siri S.; Kolb, Gregory J. (2010-05-01). "Development and Evaluation of a Prototype Solid Particle Receiver: On-Sun Testing and Model Validation". Journal of Solar Energy Engineering. 132 (2). doi:10.1115/1.4001146. ISSN 0199-6231.
- Ho, Clifford; Christian, Joshua; Yellowhair, Julius; Armijo, Kenneth; Kolb, William; Jeter, Sheldon; Golob, Matthew; Nguyen, Clayton (2016). "Performance evaluation of a high-temperature falling particle receiver". Volume 1: Biofuels, Hydrogen, Syngas, and Alternate Fuels; CHP and Hybrid Power and Energy Systems; Concentrating Solar Power; Energy Storage; Environmental, Economic, and Policy Considerations of Advanced Energy Systems; Geothermal, Ocean, and Emerging Energy Technologies; Photovoltaics; Posters; Solar Chemistry; Sustainable Building Energy Systems; Sustainable Infrastructure and Transportation; Thermodynamic Analysis of Energy Systems; Wind Energy Systems and Technologies. doi:10.1115/es2016-59238. ISBN 978-0-7918-5022-0.
- "Generation 3 Concentrating Solar Power Systems (Gen3 CSP)". Energy.gov. Retrieved 2023-07-04.
- "Generation 3 Concentrating Solar Power Systems (Gen3 CSP) Phase 3 Project Selection". Energy.gov. Retrieved 2023-07-04.
- Kraemer, Susan (2023-02-18). "Sandia breaks ground on its Gen-3 particle-based CSP tech demo". SolarPACES. Retrieved 2023-07-04.
- Xiao, Gang; Guo, Kaikai; Ni, Mingjiang; Luo, Zhongyang; Cen, Kefa (2014-11-01). "Optical and thermal performance of a high-temperature spiral solar particle receiver". Solar Energy. 109: 200–213. Bibcode:2014SoEn..109..200X. doi:10.1016/j.solener.2014.08.037. ISSN 0038-092X.
- Wu, W.; Amsbeck, L.; Buck, R.; Uhlig, R.; Ritz-Paal, R. (2014). "Proof of Concept Test of a Centrifugal Particle Receiver". Energy Procedia. 49: 560–568. doi:10.1016/j.egypro.2014.03.060.
- Flamant, Gilles; Hernandez, Daniel; Bonet, Claude; Traverse, Jean-Pierre (1980-01-01). "Experimental aspects of the thermochemical conversion of solar energy; Decarbonation of CaCO3". Solar Energy. 24 (4): 385–395. Bibcode:1980SoEn...24..385F. doi:10.1016/0038-092X(80)90301-1. ISSN 0038-092X.
- Wu, Wei; Trebing, David; Amsbeck, Lars; Buck, Reiner; Pitz-Paal, Robert (2015-08-01). "Prototype Testing of a Centrifugal Particle Receiver for High-Temperature Concentrating Solar Applications". Journal of Solar Energy Engineering. 137 (4). doi:10.1115/1.4030657. ISSN 0199-6231.
- Center (DLR), Institute for Solar Research of the German Aerospace. "DLR - Institute of Solar Research - DLR's innovation CentRec® offers new cost reduction opportunities". www.dlr.de. Retrieved 2023-07-04.
- Flamant, Gilles; Hernandez, Daniel; Bonet, Claude; Traverse, Jean-Pierre (1980). "Experimental aspects of the thermochemical conversion of solar energy; Decarbonation of CaCO3". Solar Energy. 24 (4): 385–395. Bibcode:1980SoEn...24..385F. doi:10.1016/0038-092X(80)90301-1.
- Hunt, A. J. (1979-04-01). "New solar thermal receiver utilizing a small particle heat exchanger". OSTI 5980027.
{{cite journal}}
: Cite journal requires|journal=
(help) - Calderón, Alejandro; Barreneche, Camila; Palacios, Anabel; Segarra, Mercè; Prieto, Cristina; Rodriguez‐Sanchez, Alfonso; Fernández, Ana Inés (2019). "Review of solid particle materials for heat transfer fluid and thermal energy storage in solar thermal power plants". Energy Storage. 1 (4). doi:10.1002/est2.63. hdl:2445/175822. ISSN 2578-4862. S2CID 181863403.
- Siegel, Nathan P.; Gross, Michael D.; Coury, Robert (2015-08-01). "The Development of Direct Absorption and Storage Media for Falling Particle Solar Central Receivers". Journal of Solar Energy Engineering. 137 (4). doi:10.1115/1.4030069. ISSN 0199-6231. S2CID 110006586.
- Knott, R. C.; Sadowski, D. L.; Jeter, S. M.; Abdel-Khalik, S. I.; Al-Ansary, H. A.; El-Leathy, Abdelrahman (2014-06-30). High Temperature Durability of Solid Particles for Use in Particle Heating Concentrator Solar Power Systems. American Society of Mechanical Engineers. doi:10.1115/ES2014-6586. ISBN 978-0-7918-4586-8.
- Ho, C. K.; Christian, J.; Yellowhair, J.; Jeter, S.; Golob, M.; Nguyen, C.; Repole, K.; Abdel-Khalik, S.; Siegel, N.; Al-Ansary, H.; El-Leathy, A.; Gobereit, B. (2017). "Highlights of the high-temperature falling particle receiver project: 2012 - 2016". Solarpaces 2016: International Conference on Concentrating Solar Power and Chemical Energy Systems. AIP Conference Proceedings. 1850 (1): 030027. Bibcode:2017AIPC.1850c0027H. doi:10.1063/1.4984370.
Further reading
- Mills, Brantley; Ho, Clifford; Schroeder, Nathaniel; Shaeffer, Reid; Laubscher, Hendrik; Albrecht, Kevin (2022). "Design Evaluation of a Next‐Generation High‐Temperature Particle Receiver for Concentrating Solar Thermal Applications". Energies. MDPI. 15 (5): 1657. doi:10.3390/en15051657.
- González-Portillo, Luis; Albrecht, Kevin; Ho, Clifford (2021). "Techno-Economic Optimization of CSP Plants with Free-Falling Particle Receivers". Entropy. MDPI. 23 (1): 76. Bibcode:2021Entrp..23...76G. doi:10.3390/e23010076. PMC 7825578. PMID 33419200.
- Röger, Marc; Amsbeck, Lars; Gobereit, Birgit; Buck, Reiner (2011). "Face-Down Solid Particle Receiver Using Recirculation". Journal of Solar Energy Engineering. ASME. 133 (3). doi:10.1115/1.4004269.
- Buck, Reiner; Sment, Jeremy (2023). "Techno-economic analysis of multi-tower solar particle power plants". Solar Energy. Elsevier. 254: 112–122. Bibcode:2023SoEn..254..112B. doi:10.1016/j.solener.2023.02.045. S2CID 257592663.